Climate change is complicating revegetation
By John Fitzsimmons
Revegetation is a complex specialist subject built on knowledge and experience of many interconnected fields. It is now an integral process in repairing damage to landscapes following fire or damaging weather events, post-mining or other industrial activity, and re-connecting disrupted ecosystems. Site management and plant selection have been major components of revegetation programs. However, climate change is now appearing as a long-term factor in plant selection and ultimate project outcomes.
Once plant selection for revegetation projects would have been a relatively simple process based on ‘suitable’ genus, species and, often, indigenous provenance. That is still largely the case. However, over recent years, climate change has been shown to have some effects on plants that may have to be considered to realise long-term success. Plants are responding to changing growing environments in different ways – as individual organisms and as populations. A practical reference to this trend was published in last month’s Hort Journal Australia (‘Adapting fruit trees to a changing climate’) by Gabrielle Stannus.
To follow the emerging story it is handy to revisit our knowledge of plant physiology and genetics (but it’s easier to leave the detail to the high-end specialists in these fields). It also seems to be agreed that a lot more detailed research is required, certainly involving a wider range of plant types and species, to further understanding and help decision making. This article is just an introduction to the current observations as a broad and basic summary.
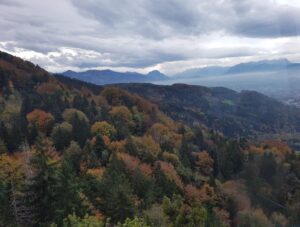
One observation of note is the high value of plant collections, seed banks and remnant populations. They are vital reference points and benchmarks of ‘what was once’ to establish the movement to ‘what we have now’ and ‘what is likely in the future’. As a comparison, just think of major human migrations – out of northern Europe escaping religious persecution, from various regions following war, or out of Ireland because of the potato famine. The emigrants took certain habits, customs and attitudes with them and adapted them to new places; and yet, the societies and places left behind continued to evolve in a different way in response to other factors. Upon returning ‘home’ they often indeed found “the past is a foreign country”.
Plant adaptation
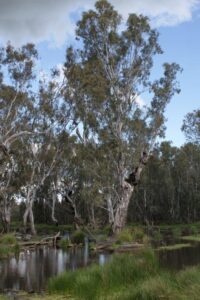
Plants adapt to various stimuli – seasonal variations, climate trends, and grazing by herbivores or insects, for example. The responses can be seen in individual plants and in populations. The potential for evolutionary changes depends on the strength of selection exerted by environmental changes and also on the number of generations that have passed.
Researchers have identified how plant responses to climate change can be classified into ‘escape’, ‘avoidance’ or ‘tolerance’ mechanisms. Research has demonstrated for example, how advanced flowering can help plants escape future droughts, how increased root biomass or rooting depth can also help them survive droughts, and how they can resist drought stress by physiological adjustments such as changes in their osmotic potential.
Research (Rauschkolb et al., Evolution of plant drought strategies and herbivore tolerance after two decades of climate change) has observed that “ongoing global warming, coupled with increased drought frequencies, together with other biotic drivers may have resulted in complex evolutionary adaptation. In three out of four studied species, evidence was found that descendants had evolved shorter life cycles through faster growth and flowering. Shifts in the osmotic potential and leaf dry matter content indicated that descendants also evolved increased drought tolerance.” Data suggested that adaptive evolution was underlying some of the observed phenotypic changes.
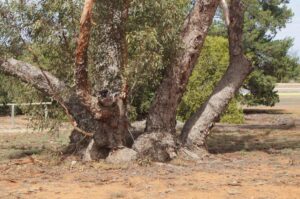
“In summary, our study revealed evolutionary changes in plant populations over the last decades that are consistent with adaptation of drought escape and tolerance as well as herbivory avoidance.”
In addition to climate change impacts, “insect herbivory is another important stress and driver of evolutionary changes in plants, Futuyma & Agrawal, and other researchers found.” They also pointed out the insect populations and their pressures on plants “is also strongly affected by climate change. Ectothermic organisms (which cannot produce their own body heat) such as insect herbivores and arthropods, including mites, are directly influenced by climate warming. It has been suggested that increased drought could lead to more insect outbreaks because the relative nitrogen content in leaves is elevated. However, the drivers and interactions are an area where substantially more research has been called for – especially because “we know much less about long-term trends in insect herbivory than about trends in climate” (Turcotte et al., 2014).
Climate change influences various species interactions, but it has been described as “challenging” to assess just how these interactions are affected. Interacting species will differ in their migratory, phenological, and fitness responses to climate change, which can affect distribution, abundance, and timing of interactions. Some recent herbarium studies have found increased levels of insect damage on herbarium specimens during the 20th century (Meineke et al., 2019, 2021), suggesting that insect herbivory may have changed in parallel with climate in some geographic regions. It has also been suggested that the advancement of flowering could be an adaptive strategy for plants to escape both climate change and insect herbivory.
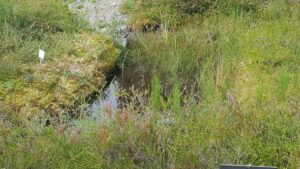
Climate change effects
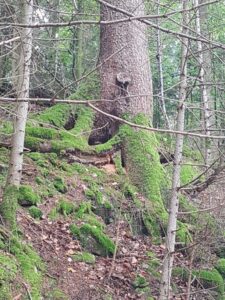
There should be little doubt about the reality of climate change. A recent research project compared multiple plant species on sites in France (approx. latitude 43oN) and in Belgium (approx. latitude 50oN). It was found that the source populations of the species studied underwent significant climate changes over the last decades. For the French/Mediterranean species, average temperatures in March–July had increased by 1.6°C and precipitation increased about 5.5 mm per year through 1991–2020 compared with the baseline (1961–1990), leading to a slight increase in drought. In Belgium, the average temperatures between 1991 and 2020 increased by about 0.9°C in the area of origin of one species and by 1.3°C in the area of another; precipitation in spring and summer decreased by 80mm and 38mm per year, respectively.
Evidence was found in the above project that descendant populations of three species evolutionarily shortened their life cycles, presumably in response to climate change, during a period of only 21–26 years. Shortened life cycles may allow plants to escape increasingly frequent summer droughts and potential insect outbreaks. Plants realised this through the ‘escape’ strategies of rapid seedling growth, earlier flowering onset and/or shifts in resource allocation. Evolutionary changes were also recognised, indicating the evolution of greater drought and herbivory tolerance through increased phenotypic plasticity in the descendant plants.
The study demonstrated the power of historical comparisons between banked seeds and current populations for studying rapid evolutionary changes.
In Australia, we often think first of the heat effects of climate change. However, in high-elevation and high-latitude systems, researchers Fyfe et al., reported that climate change is rapidly reducing winter snowpack and accelerating spring snow melt, which can expose plants to spring frost they may not have experienced historically and contribute to the decline of native plant species.
Researchers Anderson and Song noted “the capacity of plants to adapt to the direct and indirect consequences of climate change will influence extinction risks, agricultural and environmental sustainability, and food security. In the face of persistent and worsening climate change, it has become crucial to investigate how natural populations and communities respond to novel environments.”
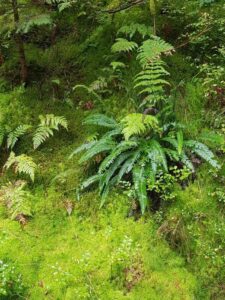
They reported that research showed several clear patterns that have emerged, including “the distributions of many species have shifted to historically cooler regions in poleward and upslope directions. These shifts have involved local extinctions and population contractions at the warmer range edges as well as range expansions into historically cooler regions at poleward latitudes and upslope elevations. Many species also now emerge and reproduce significantly earlier in the year – “likely a biological response to shortened winters, earlier onset of the growing season, and prolonged droughts”.
Expert reviews and summaries say (plant) populations risk declines if they do not have enough genetic variation to adapt to new pressures and if immigration is insufficient to introduce alleles adapted to warmer conditions. Genetic trade-offs across traits could constrain adaptive evolution, and many species will fail to migrate fast enough through highly fragmented landscapes to keep pace with climate change.
They add that species that can successfully extend their ranges into previously unoccupied habitats “will experience novel selective pressures to which they are not currently adapted”.
Anderson & Song’s review said, “We still have a limited understanding of how adaptive evolution, phenotypic plasticity, and gene flow will interact to influence population persistence under climate change. By identifying the evolutionary consequences of climate change, we can generate more robust predictions about extinction risks while identifying populations to prioritise for conservation actions.”
- Phenotypic plasticity: The changes in an organism’s behaviour, morphology and physiology in response to a unique environment variation. Phenotypic plasticity includes types of environmentally induced changes that may or may not be permanent.
- Gene flow: The transfer of genetic material from one population to another. Gene flow is an important mechanism for transferring genetic diversity among populations. Gene flow can be ‘assisted’.
- Genetic drift: The change in the frequency of an existing gene variant (allele) in a population due to random chance or the random variation in allele frequencies between generations in finite populations. Genetic drift is important in conservation biology as it is one of the factors that determines the minimal viable population size of a species.
Under rapid environmental change, gene flow could spread beneficial mutations, enhance genetic variation, and introduce pre-adapted genotypes. If populations have diverged genetically in response to climatic variation, then a genetic variation may already exist, enabling continued adaptation to climate change.
Gene flow from populations adapted to hot and dry climates into populations that historically experienced cooler conditions could facilitate adaptation to changing climates. Alternatively, gene flow could counteract local selection and constrain adaptation to climate change if gene flow occurs in the opposite direction (e.g., from high to low-elevation populations). When will gene flow ‘promote’ versus ‘restrict’ adaptive responses to climate change? How much gene flow is needed, and from which populations? Will natural levels of gene flow be sufficient, or will conservationists need to adopt practices such as assisted gene flow (Aitken & Whitlock, 2013)? Such questions underpin the growing need for more research.
The value of collections
Herbarium and other seed and plant collections are invaluable for characterising and comparing historical distributions, phenology, and trait values of many species. Studies have utilised herbarium records to show changes in geographic ranges in response to climate change and quantify phenotypic changes through time, in one case for a species* across its native range over the course of 200 years (*Arabidopsis thaliana, Thale cress, a winter annual brassica with a short lifecycle and a small genome [the first plant to be DNA sequenced] and therefore popular model organism in plant biology and genetics). These collections also enable researchers to readily compare how different species have responded to anthropogenic (caused by human intervention) climate change. Using data from herbarium records for 141 species it has been shown that species flowering in the spring are highly responsive to changes in temperature.
In addition to helping predict species interactions in the face of climate change, the maintenance of sufficient examples of genetic variation is essential for adaptation to rapid climate change.
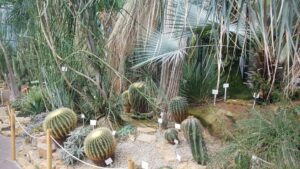
Landscape genomics is one way of testing the relationship between genomic variation and environmental heterogeneity among natural populations. Two approaches have been used to identify candidate genes involved in environmental adaptation. One involves scanning the genome of populations in different habitats, and the other is genome-wide association study between molecular markers and environmental variables, which are treated as phenotypes. These two methods require different sampling strategies. Integrating both methods can help identify the genetic basis of climate adaptation, especially for tree species with long life cycles.
Despite much research identifying candidate genes associated with various traits related to climate conditions, such as drought and thermal stress, little has evaluated the function of candidate genes that may influence climatic adaptation. Very little is known about the molecular mechanisms involved in climate adaptation.
Under current climate change trends, increasing average temperatures are causing hotter summers and more temperature variability. Phenotypic plasticity can help plants overcome the negative effects of temperature variability and allow them to rapidly adjust traits to adverse conditions. Research suggests that a plant’s developmental stage during heat stress is a key determinant of its response.
All images supplied by John Fitzsimmons